[authors: Ben Daee and Sadegh Khosravi]
Introduction
The clock is ticking on fossil fuels. Protecting the environment by “going green” is not only on the near horizon of the power generation industry across the world, but the initiative has been adopted by many other industries including auto, high-tech, and manufacturing. Renewable energy sources constitute almost 63% of electricity production in Canada (15% in the US). While solar power only accounts for less than 0.5% of the total power generation in Canada (0.9% in the US)[1], it is the fastest growing source of electricity worldwide among all types of renewable energies. There are many reasons why large-scale solar photovoltaic (PV) panels have not become widespread in North America; some obstacles could be low efficiency, high maintenance cost, politics, etc. On a small scale though, PV panels (solar panels) are being installed on more residential and commercial roofs with over 50% annual growth[2] since 2010.
Adding solar panels on the roof of a building has become less expensive than ever and it is made possible by mass production and tariff exemptions of the imported parts. Tax incentives, profit of power buyback programs, and ever-rising electrical bills justify the cost of solar panel utilization for home and business owners. Cost-benefit analysis and the return on “solar investment” look attractive on paper over a 20-year term; however, the underlying risks of roof-mounted solar panels are typically not well known to owners and insurance companies.
On a larger scale, solar farms (i.e., series of ground-mounted solar panels) are a fast-developing industry that have witnessed a boom over the past decade and have become one of the common sources of clean energy. Although solar farms are cost effective in certain geographies, they require several acres of land and proximity to a power grid. Also, soil conditions, the number of sunny days, risk of wildfire, maintenance issues, frequency of storm events and legal repercussion can shorten the facility’s lifespan and/or its ultimate profitability[3].
This article aims to scrutinize the risks of roof-mounted and ground-mounted solar panels. Forensic review of several cases has revealed that all risks associated with solar systems may not be fully understood by the owners, insurers, or other involved parties. The decision of utilizing a roof-mounted solar system, or determining the location of solar farms, is typically made by the owners and is conducted by the product supplier who often provides engineering consultation for the project. Adding new components on an existing roof may alter the exposure of the building to new environmental loads while structural aspects of the project are sometimes overlooked due to the complexity. Similarly, solar farm projects have long-term impacts on the environment that should be thoroughly contemplated at the design stage; however, solar energy is still significantly cleaner and less risky than other sources of power generation.
General Risks of Solar Panels
Fire is the greatest concern of solar panel development. While PVs cannot start a fire themselves, installation malfunctioning and overheated components can. The other concern is that even in the case of suppressing a PV related fire, firefighters are exposed to the risk of electrical shock.
Placing solar arrays on roofs may pose additional risks of roof collapse or water intrusion. Solar panels are subject to great fluctuations in temperature, moisture exposure, and freeze-thaw cycles. Direct exposure to the outdoor environment accelerates wear and tear and increases the likeliness of component failure. Since there is currently no uniform standard in North America to provide guidance for the structural design, installation, and maintenance of solar panels, the risks vary case by case depending on the project characteristics including size, location, and installation approach.
For residential properties certain risks arising from rooftop solar panels such as damage caused by peril (e.g., fire, windstorm, and hail) may be covered under a standard home policy since they are typically considered as part of the property. However, for commercial buildings the business interruption loss resulting from solar panel failure may not be covered under a standard policy. Hence, commercial buildings are typically at a potentially greater risk of damage as a result of roof-mounted solar panels than residential buildings, although statistical studies in this area are limited.
Solar farms share similar environmental risks with roof-mounted solar panels, e.g., hail, freeze-thaw, and wind damage. However, they are exposed to additional losses such as frost heave, foundation failures, and significant movements due to variation in soil moisture or flooding. Solar farm development can pose a risk of wildfire to an extended area although there are certain ways to mitigate such risk. Given the amount of required land for a solar farm, aesthetics, proximity to residential areas, and the potential impact on wildlife risk can also be concerns during solar farm development.
Fire Hazard
The number of reported fire incidents involving buildings with roof-mounted PV systems and solar farms has sharply increased recently as utilization of solar panels has risen exponentially [4] in the last decade. Solar panels are not typically the source of fire. Electrical arcing due to improper installation, faulty wiring, or insufficient insulation are often the main causes of fire. Inverters and junction boxes should be regularly monitored to avoid the risk of overheating, arcing, and short circuits. The conduit leading from PV panels to an inverter may remain live with direct current even after the main service panel has been shut off. As such, firefighters are at the risk of electric shocks which make the fire suppression more challenging.
PV equipment can combust with intense heat and flames that lead to wildfires. Systemic overheating damage to solar cells is easy to overlook during a basic visual assessment, and risk mitigation of sweeping fire should be considered at the design stage. To reduce the risk of fire in solar farms, accurate design, installation by professionals, a regular inspection regime, and technological development are critical components that typically lead to a safe green development.
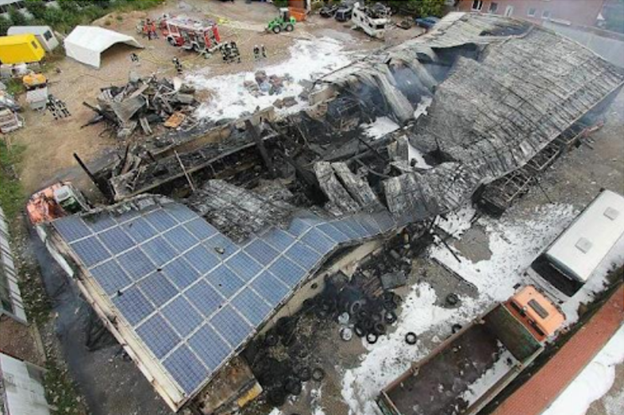
Figure 1 - Fire in a factory in Norderney Germany, 8/2013. Damage cost totaling millions of euros (IEA) (Source: https://solarity.cz/blog/fire-hazards-and-mitigation-in-photovoltaic-systems/)
Environmental Risks
Snow Load
North American building codes do not currently include explicit provisions intended for snow loads on roofs with installed solar panels. In the absence of specific structural standards, the engineering judgment is left to the designer who must account for the amount and distribution of snow load on a flat (low slope) roof. Nonetheless, Minimum Design Loads and Associated Criteria for Buildings and Other Structures Standard (ASCE-7)[5] requires a saw-tooth load pattern—rather than a uniform snow load—to be considered for design of roof elements where an irregular roof surface is present. When several rows of solar arrays are installed on a flat roof, snow may accumulate in a saw-tooth pattern as the spaces between the solar arrays can be filled with snow drifts as a result of obstructions. This pattern of snow accumulation on PV systems has been witnessed and reported in commercial roof damage subsequent to a heavy snowfall in certain geographical regions of North America. This pattern alters the load distribution and can increase the amount of snow load up to 25% in comparison to roofs without solar arrays. Moreover, non-uniform loading intensifies the internal stresses of certain roof elements which were not anticipated in the original design and is potentially enough to even cause damage to a well-designed roof absent of added solar panels.
For residential sloped roofs where PV panels are flush-mounted, the alteration in the original amount of snow load is not typically critical since the roof geometry remains relatively unchanged. However, the panels convert the distributed snow load to several concentrated loads at their anchor points. Since the panels are typically installed on only one or two roof facets, the structural design of the roof framing needs to be rechecked for such load combinations. Some studies have shown that solar panels on sloped roofs may reduce the amount of snow load by 5% as result of their heating and thermal absorption characteristics. However, this may lead to a higher likelihood of formation and retention of ice on the roof, which, in turn, may cause other problems.
Snow accumulation and frost formation on ground-mounted solar panels can reduce the performance of the PV panels. Spaces between each row, and the minimum distance from the bottom of panels to the ground, can reduce the risk of damages to the PV system due to the accumulation of snow.[6] In general, adverse risk of snow on ground mounted PVs is less than that on roof-mounted systems.
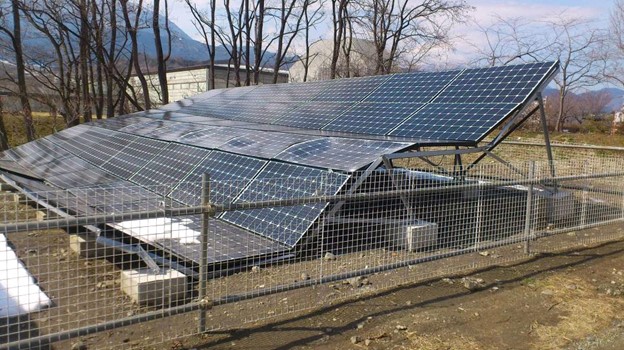
Figure 2 - Panels damaged from accumulated snow that has since melted away (Source: https://www.aquasoli.com/blog/snow-load-damages-reported-in-japan/)
Wind Load
Wind flow pattern on PV panels can be complex. Angled solar arrays act like a sail and alter the turbulence and wind pressure distribution on the supporting roof or structure. As such, the solar racks and the supporting structure should be designed for the corresponding wind loads. If PV panels are added on the roof of an existing building, the roof components should be structurally redesigned for the new wind loads in combination with other loads for which they were not originally designed.
Wind loads on the ground-mounted photovoltaic (PV) arrays are usually critical, particularly if the patterns of wind flow around the arrays (vortex shedding) create a matching vibration with the natural frequency of the structure. Research has shown that vortex shedding phenomenon usually dominates the fluctuating load not on the first row but on the second, or other rows of arrays[7]. Designs based solely on the code requirements do not guarantee long-term reliability of solar farms.
For large and high-profile projects, wind tunnel testing is the only reliable method to determine the wind load on roof-mounted solar panels as other approaches may lead to over-designed or under-designed results. For mid-size projects, Computational Fluid Dynamics (CFD) analysis is an appropriate approach wherein results can be compared with the available recommended values of engineering guidelines and deployed for the design. For smaller projects, though, using simplified approaches could be an option as long as an experienced consultant evaluates the structural performance of the existing building and considers all loading scenarios which are likely to occur. Such approaches, although common, sometimes result in either oversimplification or conservatism in structural design which can be risky or costly.
Similar to snow load, wind load is typically not critical for flush-mounted PV systems on sloped residential roofs if the system’s total height is limited to a few inches. In this case, the air plenum underneath the panels tracks the pressure above the panel and does not create considerable wind uplift on the roof depending on the size and spacing of the panels. There is a common misconception that panels situated at angles of 10-15 degrees cannot significantly change the wind load applied on a roof. Wind engineering and forensic studies have shown the opposite—roof collapses after the addition of solar farms are not uncommon.
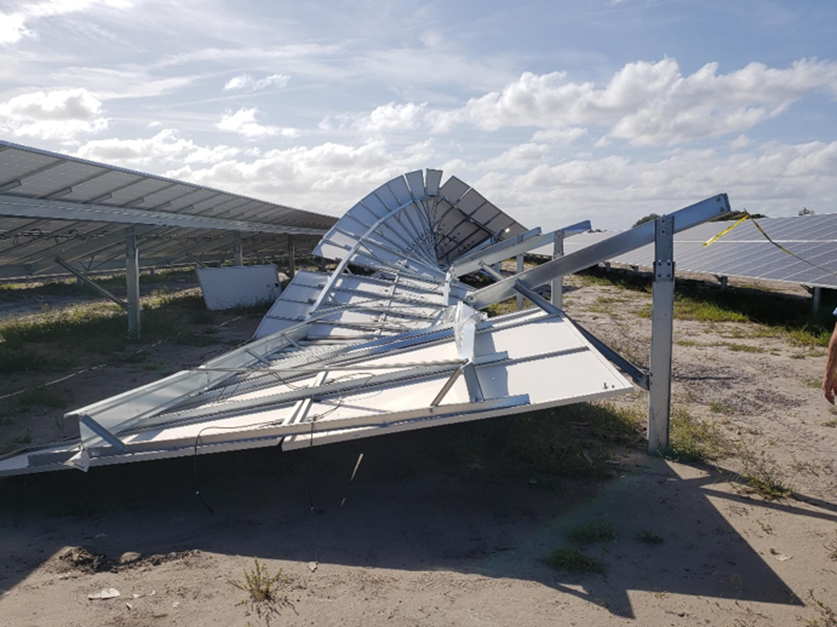
Figure 3 - The panels lying on their side in the background came off during a storm, were blown around, and were found in the water on the site. (Source: https://dailyhaymaker.com/coastal-nc-storm-damage-raises-even-more-questions-re-solar-farms/)
Ice Load
Many engineering standards prescribe consideration of ice load for ice-sensitive structures. ASCE 7 defines ice-sensitive structures as “structures for which the effect of an atmospheric icing load (i.e., freezing rain) governs the design of a portion or the entire structure.” For structures such as steel power towers, guyed masts, ski lifts, etc., radial layers of ice can form around the structural elements which elevates their weight, internal stresses, and wind-exposed faces. As previously discussed, there is no generally acceptable structural standard for the design of solar panels. However, forensic experience and site inspections conducted after ice storms showed that solar racks can be categorized as ice-sensitive structures. This not only affects the design of solar racks (supporting frames) but can change the ultimate load applied on the supporting structures/ foundation system.
Frost-Heave
PV systems are typically designed for a lifespan of 20-25 years; however, in cold regions the effective life expectancy of ground-mounted systems may be shorter due to some aggressive environmental conditions. Frost-heave may affect the power generation and even stability of solar racks. In sub-zero temperatures, water in the soil freezes, and the volume of the soil around the footings, e.g., micro piles, increases. This results in upward movement of the solar racks. Depending on the type of the soil, this cyclic frost-heave movement can occur at a rate ranging from 1/64” to 3/4” of an inch per day for an embedded foundation in certain weather conditions.[8] The risk of frost-heave is higher in clay and silt and lower in sand and gravel.[9]
Frost-heave results in structural deflection and changes in the angle of solar panels. Non-uniform deflections of the footing system may cause failure or deformation of connections, racking systems, disconnection of conductors, and/or grounding in the frozen soil. Proper design of PV systems for possible impact of frost heave can reduce the risk of damages. For instance, the footings or micro piles can be designed under the frost line per the building codes recommendation; however, determining the uplift force by frost-heave on piles is challenging although 15 psi is a typically recommended value[10]. Nonetheless the piles can be pushed out of the ground even if driven under the frost line.
Gravity Load
Mechanically attached PV systems on sloped residential roofs typically weigh 2-4 pounds per square foot. This is not a considerable amount of weight as an intact well-designed roof is expected to withstand this additional load with limited or no modification. However, alteration in structural systems or loads of an existing building may require code upgrades that may affect the original design of the building due to more stringent requirements. Gravity loads of ballasted PV systems on flat roofs are considerable and may impose an additional 5-30 pounds per square foot on the roof framing for which a detailed structural analysis is required.
The main challenge for both methods of installation is that the weight of solar panels is not uniformly distributed on the roof and may have critical local effects on certain structural elements. If detailed drawings for the location of arrays are not produced, the actual loads on the roof will differ from the design loads as repositioning of solar arrays on the roof in the construction stage is likely.
Water Intrusion and Ponding
Solar panels on a sloped roof are attached to the roof framing using mechanical fasteners. For existing buildings, this means that numerous holes must be drilled into the roofing system. The anchor holes are typically sealed to prevent water leakage into the building. However, the performance of the seals is a factor of installation accuracy and long-term behavior of the sealant material’s exposure to environmental elements. Vibration of the panels due to wind forces, as well as thermal expansion/contraction cycles, may result in seal breaks and water intrusion.
On flat roofs, solar racks may disturb the drainage path of water to the roof drains depending on their supporting configuration. Ballasted solar racks are equally spaced in parallel rows on the roof and may result in rainwater or snow melt accumulation between the modules. Water ponding can occur in these strip regions which may lead to accelerated delamination, cracking, and sagging of the roof and, ultimately, water intrusion to the building.
Conclusion
Installation of solar panels on flat or sloped roofs may alter the roof geometry and its capacity, especially when factoring in exposure to environmental loads. Snow load, ice load, wind load (plus wind on ice), additional dead load, water ponding, drainage obstruction, and water intrusion not only influence the structural design of buildings but may also affect their long-term functionality. Fire risk due to the presence of solar panels on the roof is typically higher, although risk of fire hazard can be mitigated if solar panels are installed and maintained by professionals.
The risk of damage to buildings with roof-mounted solar panels is simply higher due to the presence of the panels. Insurers may unknowingly bear a considerable portion of this risk, and the owners may not be aware of the risk exposure. Lack of a uniform engineering standard, which includes all aspects of the design and installation—particularly for flat roofs—adds more complexity to the liability arising from the solar panels.
Solar farms increase the risk of wildfire if the risk is not mitigated at the design stage. Movement of footing as a result of frost-heave may lead to permanent damage to the solar rack and power generation. Wind damage to solar farms is likely resulting from the complexity of the wind design and the effect of vortex shedding that may impose an excessive uplift load on the panels.
Simply put, the design of solar farms or roof-mounted solar panels is a multi-faceted problem which should be assessed by qualified engineers. As such, prior to deciding whether installation of roof-top solar panels is a sensible action, an owner should consider if the roof needs any structural modifications. Insurers should know if the property includes roof-mounted solar panels to consider the potential risks.
Acknowledgments
We would like to thank Ben Daee, PhD, P.Eng., PE. and Sadegh Khosravi, PhD, P.Eng, for providing insight and expertise that greatly assisted this research.
[1] Statistics Canada, The National Energy Board of Canada; The U.S. Energy Information Administration (EIA),
[3] Environmental Effects of Solar Farms, by Arthur, June 2018, https://www.ledwatcher.com/environmental-effects-solar-farms/ BY ARTHUR · LAST UPDATED JUNE 28, 2018
[4] Assessing Fire Risks in Photovoltaic Systems and Developing Safety Concepts for Risk Minimization, Office of Energy Efficiency & Renewable Energy, US department of Energy
[5] American Society of Civil Engineers
[6] Best Practices for Operation and Maintenance of Photovoltaic and Energy Storage Systems; 3rd Edition, NREL 2018. https://www.nrel.gov/docs/fy19osti/73822.pdf
[8] What is frost heave and how does it affect ground-mount solar projects, by Solar Builder, November 2019, https://solarbuildermag.com/featured/what-is-frost-heave-and-does-it-affect-ground-mount-solar-projects/
[9] U.S. Army Corp of Engineers
[10] https://www.rpcs.com/frost-heave/