One of the wonders and satisfactions of modern science has been the elucidation (usually based in genetics) of the wonders of nature that have been famously observed but not explained until the proper tools (again, usually genetic) have been developed. One of these is the ability of certain animals to grow and thrive on a diet comprising otherwise toxic substances. The koala is one example; on a different scale are insects, most noticeably monarch butterflies, that grow on milkweed known to contain cardiac glycosides inimical to life.
Recently an international group published a paper in the journal Nature entitled "Genome editing traces the evolution of toxin resistance in the monarch butterfly" that elucidated the genetic basis of this phenotype. The molecular target for these cardiac glycosides is known to be the alpha subunit of the sodium-potassium ATPase (ATPα) involved in energy generation (in higher animals associated with cardiac tissue but present in other tissues in insects). Genetic analysis of the amino acid sequence of this protein (or more accurately, the nucleotide sequence of the genes encoding them) from several species (including the monarch butterfly, Danaus plexippus) identified mutations in a particular region of the protein (the first extracellular loop, H1-H2) that is associated with target-site insensitivity (TSI), i.e., a loss of the ability for the protein to bind cardiac glycosides. Two resistance phenotypes were known, one that enables feeding on cardiac glycosides and the other that sequesters the toxin to avoid toxicity. The mutations were detected in amino acid residues at three sites (111, 119, and 122), with mutations at the 111 and 122 sites undergoing frequent parallel substitutions associated with the TSI phenotype. The 119 site showed repeated substitutions in resistant species and co-evolution with mutations at the 111 site; paradoxically, mutations at the 119 were not phenotype-specific, because they are found in non-resistant species as well. Assessing the genetic history of how these mutations arose revealed that mutation in almost all cases arose at site 119 before or in conjunction with mutation at site 122, with "repeated substitutions at the three sites [that] evolved concurrently with specialization." These authors concluded that:
The mutational paths lead to three predictions for how substitutions at sites 111, 119 and 122 affect fitness. First, the mutational paths provide stepwise fitness advantages at increasing toxin concentrations. Second, the mutational paths contribute to sequestration of cardiac glycosides through passive toxin accumulation. Third, given the ordered appearance of the substitutions, interactions between substitutions (epistasis) increase fitness and mitigate the pleiotropic fitness costs of adaptive substitutions.
These researchers then tested the effects of these mutations on resistance by using CRISPR-Cas9 mutagenesis techniques to genetically engineer Drosophila cell lines to encode ATPα having one or more of these mutations, as illustrated in this diagram:
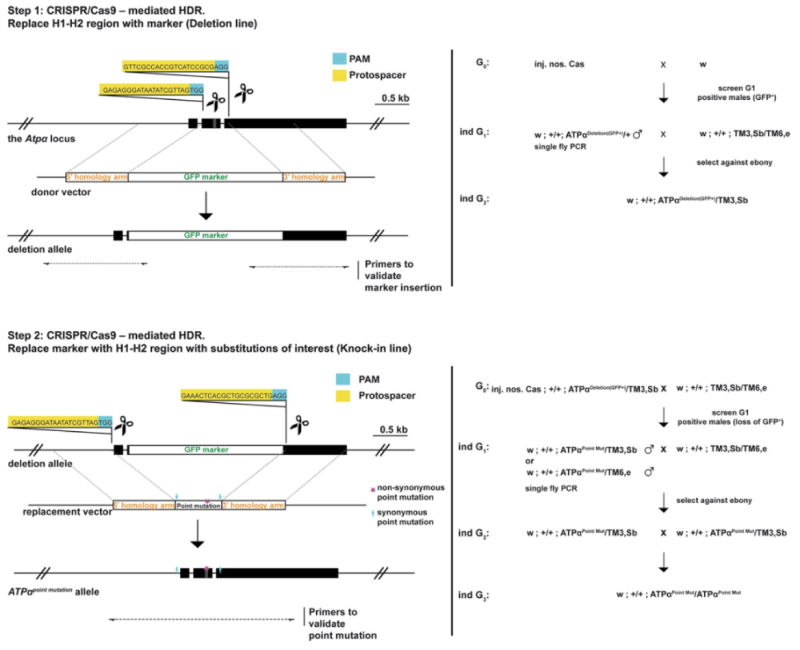
Four genotypes were tested: substitution of glutamine at amino acid 111 with either leucine or valine; substitution of serine at amino acid 119 with serine; and substitution of asparagine at amino acid 122 with histidine (producing mutants represented as LAN, LSN, VSN, and VSH, respectively; the unmutated sequence is QAN). These mutations were chosen because the mimicked the evolutionary progression of the mutations observed in the determination of sequences phylogenetically. Single site mutants were also produced (represented as QSN and QAH). Expression of the ATPα gene and sodium pump activities were unaffected in these mutants. These mutations were then used to produce "knock-in" lines of fruit flies that showed increased resistance to ouabain, a hydrophilic cardiac glycoside familiar for its use as a selective agent against human B cells in conventional hybridoma technology. In larval-adult and adult survival experiments, the LAN mutant showed increased survival in larval-adults only at low ouabain concentrations. Resistance increased in these experiments for LSN mutants, with VSN mutants behaving similarly. The VSH mutant (which is the native monarch butterfly mutant) "was unaffected by even the highest levels of ouabain in larvae and adults," a phenotype not associated merely with reductions in toxin ingestion or feeding rates. Experiments with knock-in eggs using leaves from Asclepais curassavica (milkweed) showed increased survival for the LSN, VSN, and VSH genotypes.
Physiological assessment of enzymatic activity of the mutant sodium ATPases showed a "neutral to positive" effect of TSI to ouabain. Resistance progressed (again, following the observed phylogenetic progression) from a small increase in TSI with the LAN mutant, to a ten-fold increase for LSN and VSN mutants, and a thousand-fold increase for the VSH mutation array. According to these authors, "VSH is sufficient for 'monarch [Drosophila] flies' to achieve the same degree of TSI to ouabain as the monarch butterfly, suggesting that TSI is the predominant biological mechanism for the in vivo toxin resistance observed above."
Returning to the genetics, the paper notes that "[s]ite 119 co-evolves with site 111 . . . and substitutions at site 119 always occurred before or with TSI-conferring substitutions at site 122," from which the authors speculate that "antagonistic pleiotropy and epistasis may have shaped mutational paths to resistance and TSI." To test this hypothesis, the paper reports the results of experiments using QZH and QSN mutants; the former genotype was "often the last substitution to evolve" while the latter occurs in both resistant and sensitive species. While the QSN mutant could not increase larval-adult survival at low ouabain concentrations there was a survival benefit as ouabain concentrations were increased (this effect "dropped sharply" at the higher concentrations). The results with adults were different, with QSN providing "slight" survival benefit. The last mutation to arise (N122H) gave the highest TSI phenotype but was phylogenetically contingent on substitution arising in site 119. Neurological testing showed the QSN flies were least sensitive and QAH flies the least, and the first mutant LAN was more sensitive that LSN.
The authors note that "this is, to our knowledge, the first in vivo validation of a multi-step adaptive walk in a multicellular organism, and illustrates how complex organismal traits can evolve by following simple rules."
* Department of Integrative Biology, University of California, Berkeley, Berkeley, CA, USA; Department of Biology, Center for Genomics and Systems Biology, New York University, New York, NY, USA; LAI, U1067 Aix-Marseille Université, Inserm, CNRS, Marseille, France; Department of Ecology and Evolutionary Biology, Cornell University, Ithaca, NY, USA; Department of Statistics, University of California, Berkeley, Berkeley, CA, USA; Molecular Evolutionary Biology, Zoological Institute, Biocenter Grindel, Universität Hamburg, Hamburg, Germany; Department of Entomology, Cornell University, Ithaca, NY, USA