On March 14, 2024, damage to four subsea cables resulted in widespread internet outage in 13 countries in West and Central Africa with prolonged (multiday) nationwide outages in six countries impacting millions of Africans. The importance of these subsea cables is highlighted in another recent cable failure. In early March of 2024, a cut in three undersea fiber cables in the Red Sea disrupted approximately 25 percent of internet traffic in the Middle East, Asia, and Europe. MainOne, one of the companies responsible for the West African cables, stated that likely “some form of seismic activity on the seabed resulted in a break to the cable.” Although the exact cause is still undetermined, submarine geohazards can pose significant risks to offshore infrastructure (Carter et al. 2015; Clare et al. 2017). Globally, more than 420 submarine cable systems (more than 1.8 million km of cable) connect more than 99 percent of all international digital data traffic. These cables can cost hundreds of millions of dollars to construct and have become vital infrastructure for commercial and national interests.
Geophysical hazards, such as undersea earthquakes, landslides, and sediment flows (or turbidity currents), pose significant risk to submarine cables. Submarine landslides and turbidity currents can mobilize and deposit vast quantities of sediment, orders of magnitude larger than their land-based counterparts. Submarine landslides can be on the order of 1000 cubic km in scale triggering tsunami-events (Talling 2014). Similarly, turbidity currents can transport hundreds of cubic km of sediment at high velocities (upward of 10 m/s) over thousands of kilometers (Clare et al. 2017; Talling et al. 2007). Smaller scale landslides and turbidity currents nearshore can cause significant damage to offshore infrastructure such as oil and gas facilities (pipelines, flowlines, manifolds, anchors; Niedoroda et al. 2003, 2004) as well as offshore wind facilities on the continental slope.
Due to the size and magnitude of these undersea flows, they can damage multiple subsea cables over large areas. For example, a turbidity flow associated with high discharge from the Congo River resulted in cable damage that took nearly a month to repair resulting in outages along the west coast of Africa (Talling et al. 2021). Submarine fiber optic cables may be buried in water depths less than 750 m but are typically resting on the seabed at deeper ocean depths. The small size of the cables (typically on the order of an inch in diameter) coupled with the depth of cable placement can pose significant challenges for cable repairs. Repairs to subsea cables can be in the hundreds of millions of dollars with estimates of >$400 million per year in offshore infrastructure damage associated with submarine mass movement (Mosher et al. 2010). Therefore, the placement and routing of subsea cables should be carefully considered to mitigate subsea risk.
Geophysical hazard assessment is commonly employed to support cable routing and design and uses conventional geophysical techniques, including high resolution reflection seismic and side-scan sonar (Clare et al. 2017). There are, however, new and emerging techniques in offshore geohazard monitoring that can help mitigate or better characterize geophysical risks. Multispectral multibeam echosounder (MBES) mapping methodologies have been employed to reveal seabed characteristics with greater fidelity as shown in Figure 1 that can be used to characterize geophysical hazards.
Recent advances in the deployment and processing of the latest-generation ship-based multibeam (MBES) and subbottom profiling (SBP) systems, developed by Integral’s Dan Orange and funded by the U.S. Office of Naval Research, have provided significant advances in geophysical surveying resolvability. These systems have been demonstrated to accurately map 6 to 14 in. (15 to 36 cm) diameter pipelines in up to 2650 m water depth and 1.25 in. (3.2 cm) diameter fiber optic cables in 880 to 1250 m water depth. Dr. Orange has demonstrated the ability of latest-generation SBP systems to image 8.625 in. (22 cm) diameter pipelines buried 1 m below the seafloor; this SBP can discern individual reflector layers as small as 10 cm independent of water depth.
These advances in geohazard mapping and infrastructure detection allow pipeline and cable owners to develop routing assessments that can avoid geohazards and environmentally sensitive areas with unprecedented accuracy. Improved routing can substantially reduce risks to geohazards such as turbidity flows and landslides by identifying regions of higher risk and routing cables parallel to flow routes to avoid stress on the cable. In addition to routing service, Integral supports clients by monitoring changes on the seafloor, supporting maintenance and repair activities, and identifying and mitigating environmental risks. These Integral services support the global goals of safe, sustainable, and efficient offshore activities.
Optional Figures: 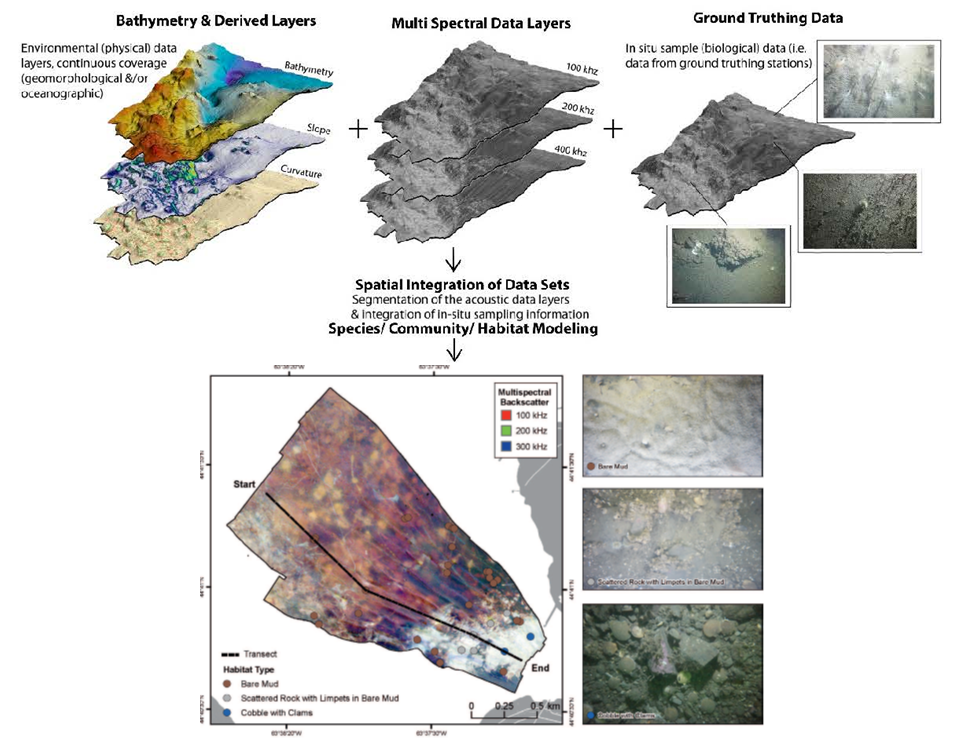
Figure 1. Bed Characterization Mapping with Enhanced MBES Methods (Brown et al. 2019).
References Cited:
Brown C.J., J. Beaudoin, M. Brissette, and V. Gazzola. 2019. Multispectral multibeam echo sounder backscatter as a tool for improved seafloor characterization. Geosciences 9(3):126. https://doi.org/10.3390/geosciences9030126.
Carter, L., R. Gavey, P.J. Talling, and J.T. Liu. 2015. Insights into submarine geohazards from breaks in subsea telecommunication cables. Oceanography 27(2):58–67. https://doi.org/10.5670/oceanog.2014.40.
Clare, M.A., M.E. Vardy, M.J. Cartigny, P.J. Talling, M.D., Himsworth, J.K. Dix, J.M. Harris, R.J. Whitehouse, and M. Belal. 2017. Direct monitoring of active geohazards: Emerging geophysical tools for deep‐water assessments. Near Surface Geophysics 15(4):427–444.
Mosher, D.C., L. Moscardelli, R.C. Shipp, J.D. Chaytor, C.D. Baxter, H.J. Lee, and R. Urgeles. 2010. Submarine Mass Movements And Their Consequences. Springer, Netherlands. pp. 1-8.
Niedoroda, A.W., C. Reed, H. Das, D. Orange, and J. Hooper. 2004. Numerical analyses of deep sea mass gravity flows. CD-ROM paper #16204. Offshore Technology Conference, Houston, TX. 9 pp.
Niedoroda, A.W., C.W. Reed, L. Hatchett, A. Young, D. Lanier, V. Kasch, P. Jeanjean , D. Orange, and W. Bryant. 2003. Analysis of past and future debris flows and turbidity currents generated by slope failures along the Sigsbee Escarpment in the deep Gulf of Mexico. CD-ROM paper #15162. Offshore Technology Conference, Houston, TX. 8 pp.
Talling, P.J., M. Baker, E. Pope, C.A. Cula, M. Cartigny, R. Faria, M.A. Clare, S. Simmons, R.S., Jacinto, M. Heijnen, and S. Hage. 2021. Novel sensor array helps to understand submarine cable faults off West Africa. Available at: https://eartharxiv.org/repository/view/2405/?trk=public_post_comment-text.
Talling, P. 2014. On the triggers, resulting flow types and frequencies of subaqueous sediment density flows in different settings. Marine Geology 352:155–182. doi: 10.1016/j.margeo.2014.02.006
Talling, P., Wynn, R., Masson, D. et al. 2007. Onset of submarine debris flow deposition far from original giant landslide. Nature 450:541–544. doi: 10.1038/nature06313